About
What is TRISH?
TRISH stands for Tree-Ring Integrated System for Hydrology.
This U.S. NSF Funded project (Office of Polar Programs) is located in two research centers: Laboratory of Tree-Ring Research, University of Arizona and Earth Systems Research Center, University of New Hampshire.
While the Yenisei River is the direct focus of the research, a broad goal is to extend these approaches, methods, and tools to other pan-Arctic regions. To assist other researchers, the team is developing a new online toolbox (TRISH) that synthesizes tree-ring signals for the heat and volume of Yenisei River and adopts non-parametric statistics for the spatial reconstruction of these climatic variables.
The online TRISH tool will be built on the existing RIMS platform hosted by the University of New Hampshire. RIMS (Regional Integrated Mapping System) is an online tool for multidisciplinary data storage, visualization, analysis, and manipulation with a focus on hydrological applications. The RIMS data pool covers a variety of themes (e.g., climate, hydrology, land cover, and demographics) and comprises over 15,000 data sets. Recently, the platform has embedded the University of New Hampshire Water Balance Model (WBM model). The WBM model and TRISH will be complemented by a hydrograph routing model (HRM) for gauged river data control.
This open-access tool can be used for both research and education, enabling societal understanding of natural hazards and environmental change induced by the Arctic amplification. The TRISH Tool website will be open to the public in Summer 2022. We look forward to your discoveries on this platform.
Yenisei River Basin
The Yenisei River (see map below) is the world’s sixth-largest river in terms of mean annual discharge (585 km³/yr) with the drain area of 2.44 million km² located on the Central Siberian Plateau. The Yenisei is the most highly developed of the great Siberian rivers that drain into the Arctic Ocean. Although the population is only a bit over 5 million people, the Yenisei River Basin (YRB) has seven reservoirs with operational capacity greater than 1 km³ and the total storage capacity of 482 km³. The YRB is located mostly in Russia but includes parts of Mongolia via the Selenga River. The climate of the YRB is subarctic in the north and strongly continental in the central parts and south. July's average temperature ranges from 46 °F (7.8 °C) in the north to 68 °F (20 °C) in the south. On the hottest summer days, the temperature can reach 105°F (40.6 °C). January average temperature ranges from −25 °F (-31.7 °C) in the north to −4 °F (-20 °C) in the south. Annual precipitation in the YRB averages 16 to 20 inches (40 to 50 cm) in the north, 20 to more than 30 inches (50 to more than 75 cm) in the central portion, and up to 47 inches (199 cm) in the high mountains in the south. Annual precipitation varies strongly with topography. Closed depressions in the upper part of the basin can receive less than 8–12 inches (20-30 cm) annually. For the basin as a whole, most of the precipitation falls in the warm months of the year. The cold temperature regime of the YRB has important consequences for the hydroclimate. An appreciable fraction of the annual discharge at the mouth of the Yenisei comes from snowmelt. Ice jams and the associated flooding occur each year. Continuous permafrost is present over about 1/3 of the basin, while 89% of the basin experiences permafrost of some type. The complexity of hydroclimatic conditions across the YRB and the extensive network of hydrological dams are both the curse and cure for hydrological modeling with tree rings. Diverse climatic signals in the ring growth variability provide a wealth of information through which we can screen large networks of tree-ring proxies for optimal reconstruction of discharge fields.
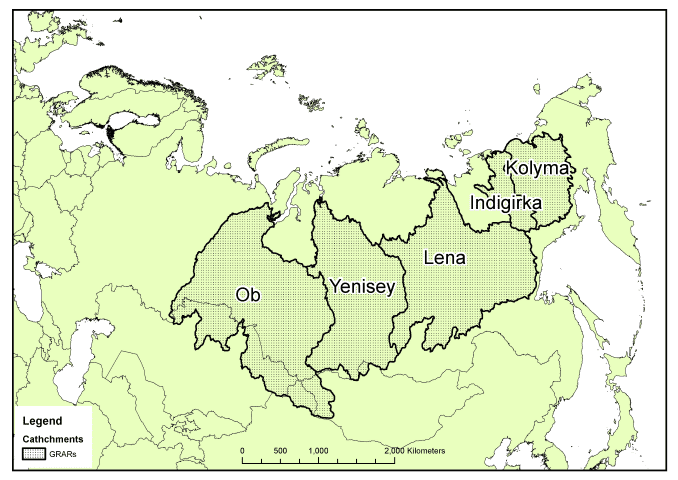
Tree-Ring Proxies
Heat transported by the Yenisei River depends on both water temperature and river discharge. The heat flux dominated by summer discharge (May-Oct) has a volume peak in June, and a heat peak in June and August. Tree-ring growth responds to weather conditions within these seasonal windows, which is most important to the Yenisei heat transport.
In fall 2019 we updated temperature-sensitive tree-ring chronologies in Northern Eurasia at the lower reaches of the Yenisei River and precipitation-sensitive tree-ring chronologies at the southern part of the Yenisei River basin. These proxies are essential to both discharge and heat flow reconstructions.
Tree-Ring Sites
The map below shows locations of the 240 tree-ring sites in the Yenisei River Basin database. Total-width chronologies are available for all sites. Sub-annual width and maximum density measurements are available for subsets of these 240 sites. We now have total-ring-width measurements for 234 of the sites. These were quality controlled to derive two networks of total-width chronologies: a) 165 higher quality sites with uniform coverage 1799-1988 and earliest data in 900 CE, and b) 30 sites specially selected for wide spacing, good sample depth, and strong common signal. The 30 site network is shown on the map in the figure below this one.
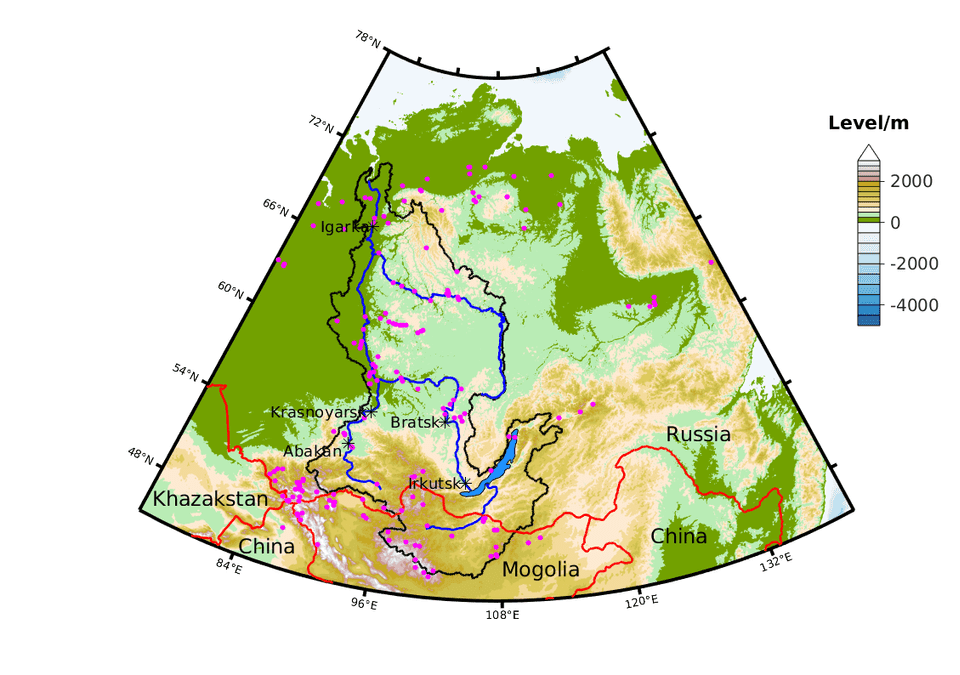
Selected Sites (with the VS-Lite Model)
The map below shows the VS-Lite network sites and locations of fall 1999 field collections. The 30 sites culled for our initial network of total-width chronologies and extended by the VS-Lite process-based growth model (Tolwinski-Ward et al., 2011) are shown as magenta triangles. Locations of 19 new sites from the 1999 field trip are shown as open magenta circles. Some circles represent multiple closely spaced sites. The 30 VS-Lite sites also comprise a pilot network we are using for developing reconstruction software. All VS-Lite sites in this network have coverage back to at least 1750, and the latest year in all chronologies was extended to 2019 with VS-Lite. Chronologies were developed with a uniform setting for detrending and other operations using program ARSTAN (Cook et al., 2007).
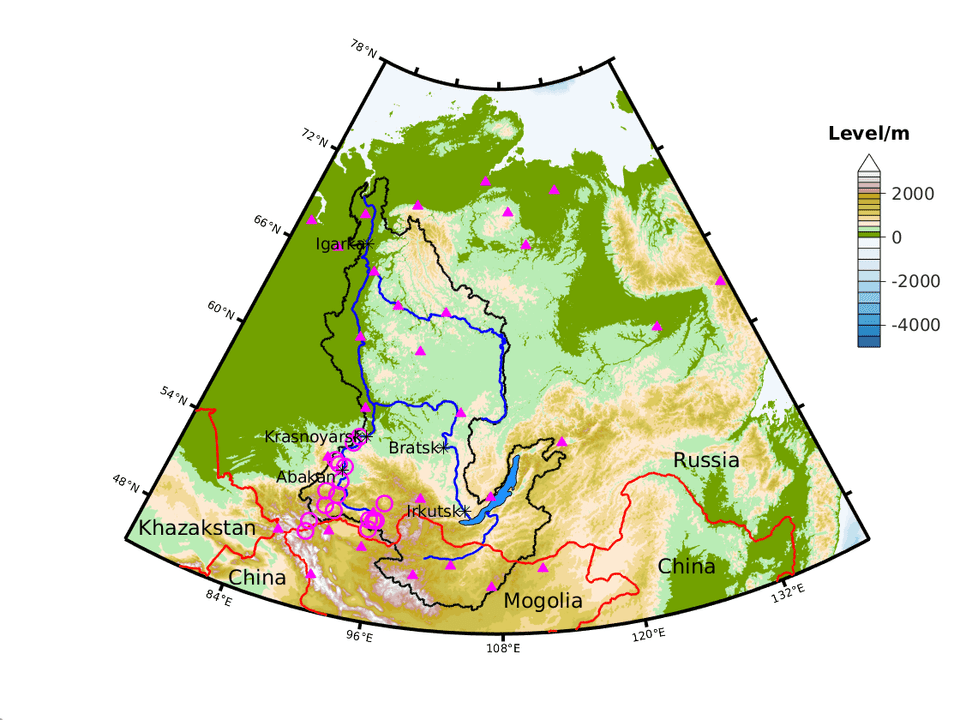
Projection of Climate Change on the YRB Discharge
The increasing concentration of greenhouse gases is projected to contribute an additional Arctic warming of about 4–7°C over the next 100 years. The warming trend strongly influences the evaporation and snowmelt regimes at the YRB, and, in combination with precipitation change, alters freshwater inflow to the Arctic Ocean. The Yenisei River contributes 22% of the average annual freshwater inflow to the Arctic Ocean, which impacts changes in sea ice (e.g., albedo) and deep ocean circulation. Global Circulation Modeling (GCM) projections suggest increasing concentration of greenhouse gases and leads to strong warming combined with precipitation increase in the YRB by the end of the 21st century (see GCM map). Many studies point to an increase of annual mean discharge (up to 15.6%) by the end of the 21st century, with a shift to an earlier seasonal peak discharge because of earlier snowmelt. The projected increase in discharge is associated with increases in both annual precipitation and annual evaporation. The regional warming potentially increases the freshwater inflow through various pathways, including increased precipitation, altered timing of snowmelt, and melting of permafrost. Changes in vegetation associated with the warming trend also trigger changes in carbon storage, alteration of runoff patterns, and forest fire that impact the YRB flux of water and energy into the Arctic Ocean.
Map showing GCM projections of changes in prescription and temperature (top) and annual total precipitation (bottom) in response to increasing concentrations of greenhouse gases. Contact us for more information about the simulation results.
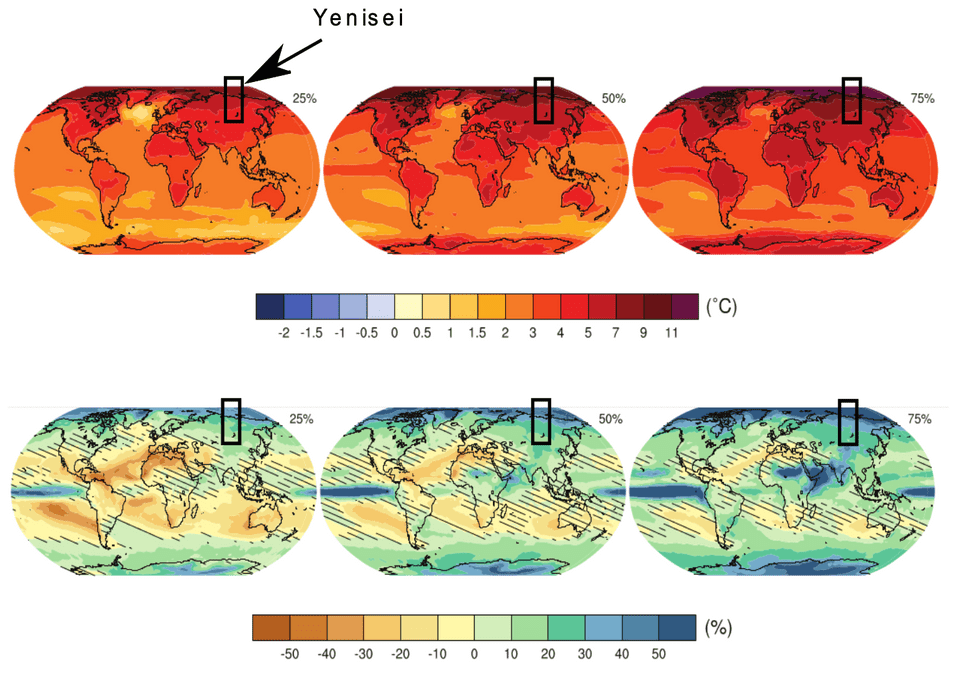
Impacts of Climate Change on the Yenisei River Basin
The general trend in annual precipitation of Siberia appears to be toward precipitation increase. Various precipitation studies investigating climatic change in Siberia show that annual precipitation increased by about 10% from the first to the second half of the 20th century, but has subsequently stabilized. Cold season precipitation, on the other hand, has been recently increasing. Although the total amount of summer precipitation has not significantly changed, heavy rainfall events have become more common in recent years (see photo). Overall, Siberia experiences an increase in winter average and maximum snow depths. The snow tendency in Siberia is similar to Canada and Alaska.
While the annual discharge of Siberian rivers flowing to the Arctic has generally been increasing in recent decades, attribution of the trend to climatic factors is extremely difficult. Streamflow trends in the YRB is particularly complex due to the thawing of permafrost (+) and increased evaporation associated with atmospheric warming (-). Additionally, the natural variance of streamflow records is heavily distorted by anthropogenic factors. Numerous large hydrological dams and reservoirs have been built since the mid 20th Century.
Any hydrological modeling in the YRB is challenged by huge uncertainties in historical climate networks due to the sparse and irregular nature of observations, which challenge the assessment of precipitation-discharge linkages. Tree-ring proxies are the independent parameters of hydrological variability in the YRB that will be tested and applied to solve these uncertainties.
Photo: Flood after very heavy rain in Krasnoyarsk city, May 2020.
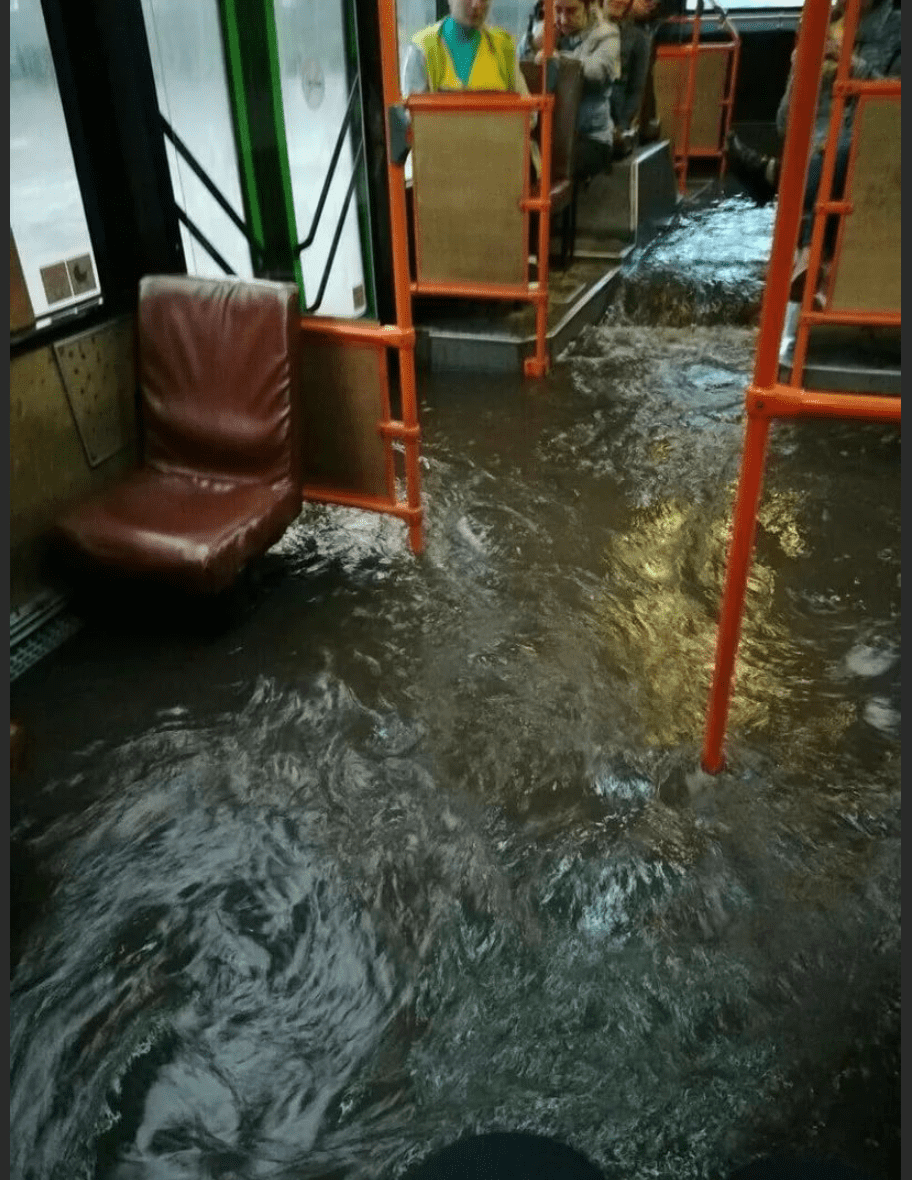